Highlights
How do Multicellular Networks become a Cooperative Unit (Supercell)?
In an orchestra many musicians cooperate to create a symphony, the “supermusician“. Individual musicians (no matter how excellent) thrown together in a room, make no symphony.
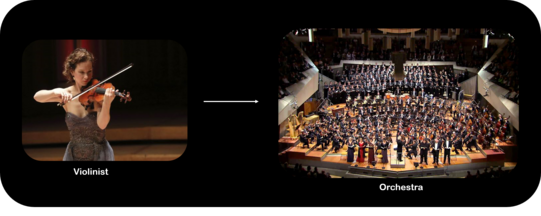
The emergence of multicellular life. We follow the formation of multicellular networks of cells from a physical perspective. I.e. we look into the physical state and the role of momentum conservation during the formation of organs.
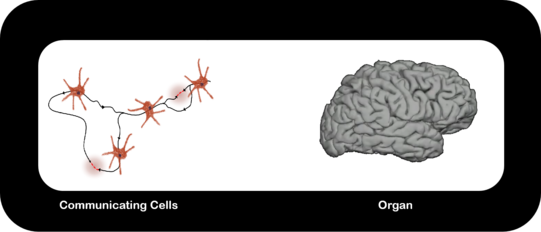
References:
- M. F. Schneider, “Living systems approached from physical principles,” Progress in biophysics and molecular biology, vol. 162, pp. 2–25, 2021, doi: 10.1016/j.pbiomolbio.2020.10.001.
Evidence of nonlinear Pulses in Interfaces
Nonlinear, acoustic pulses near phase transitions where proposed as the physical foundation of nerve pulse propagation. Our work on lipid monolayer strongly supports these prediction: Near phase transitions inter-facial pulses shift from a linear into a nonlinear regime. The threshold behavior upon excitation corresponds to the transition from liquid-expanded to liquid-condensed phase.
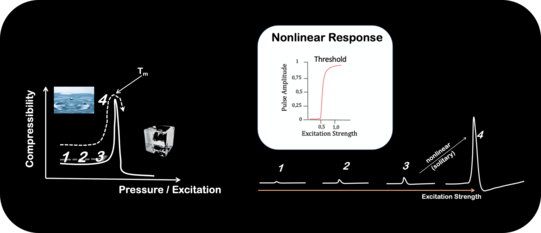
References:
- S. Shrivastava und M. F. Schneider, „Evidence for two-dimensional solitary sound waves in a lipid controlled interface and its implications for biological signalling“, Interface, Bd. 11, Nr. 97, 2014, doi: 10.1098/rsif.2014.0098.
- J. Kappler, S. Shrivastava, M. F. Schneider, und R. R. Netz, „Nonlinear fractional waves at elastic interfaces“, Physical review fluids, Bd. 2, Nr. 11, 2017, doi: 10.1103/physrevfluids.2.114804.
Momentum Conservation in Living Systems
Neglecting a conservation law violates it as laws are not obeyed by accident. Intention to violate it is not required.
The absurdities violation of energy conservation leads to are well known and we have NO reason to believe that the violation of MOMENTUM CONSERVATION leads to any less foolish pictures of nature.
Models in the life sciences, need to be reconsidered under these circumstances. In particularly ALL those dealing with communication and the propagation of signals, as momentum conservation and the 2nd law of thermodynamics leads to sound propagation, the cornerstone of all efficient propagation in media.
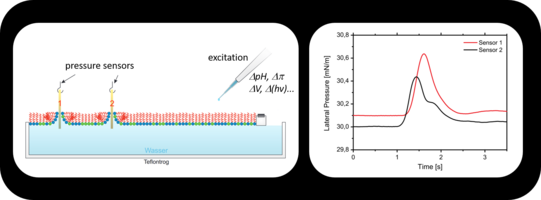
References:
- J. Griesbauer, A. Wixforth, and M. F. Schneider, “Wave propagation in lipid monolayers,” Biophysical journal, vol. 97, no. 10, pp. 2710–2716, Nov. 2009, doi: 10.1016/j.bpj.2009.07.049. [Online]. Available: https://www.cell.com/biophysj/fulltext/S0006-3495(09)01315-0
- J. Griesbauer, S. Bössinger, A. Wixforth, and M. F. Schneider, “Propagation of 2D pressure pulses in lipid monolayers and its possible implications for biology,” Physical review letters, vol. 108, 2012, doi: 10.1103/physrevlett.108.198103.
- J. Griesbauer, S. Bössinger, A. Wixforth, and M. F. Schneider, “Simultaneously propagating voltage and pressure pulses in lipid monolayers of pork brain and synthetic lipids,” Physical review E, vol. 86, 2012, doi: 10.1103/physreve.86.061909.
- S. Shrivastava and M. F. Schneider, “Opto-mechanical coupling in interfaces under static and propagative conditions and its biological implications,” PLOS ONE, vol. 8, no. 7, 2013, doi: 10.1371/journal.pone.0067524.
- B. Fichtl, S. Shrivastava, and M. F. Schneider, “Protons at the speed of sound: predicting specific biological signaling from physics,” Scientific reports, vol. 6, 2016, doi: 10.1038/srep22874.
Sharp Phase Transitions in Single, Living Cells
Phase transitions represent highly nonlinear changes in the physical properties of materials. Using high resolution fluorescence microscopy we were able to detect a phase transition within a small membrane patch of a single cell. The transition point Tm strongly depends on the pH of the system, which allows the system to switch between different physical properties (hard/soft) by chemical reactions (catalysis).
We currently study how Tm changes in the presence of different environments, proteins, drugs etc.
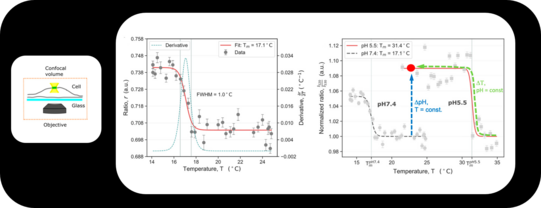
References:
- C. S. Fedosejevs and M. F. Schneider, “Sharp, localized phase transitions in single neuronal cells,” Proceedings of the National Academy of Sciences of the United States of America, vol. 119, no. 8, Feb. 2022, doi: 10.1073/pnas.2117521119.
Enzyme Activation by Sound
A pressure pulse, which is accompanied by a pH pulse, propagates along an interface. Enzymes incorporated in the interface (here Acethylcholinesterase) increase its activity by a factor of ≈ 20x upon pulse interaction.
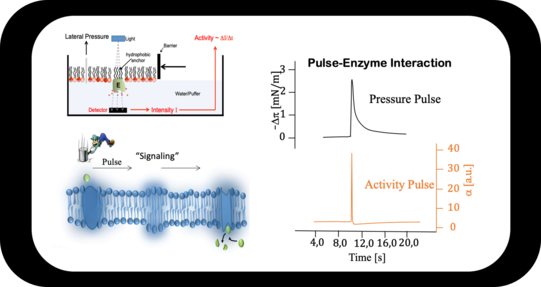
References:
- B. Fichtl, S. Shrivastava, and M. F. Schneider, “Protons at the speed of sound: predicting specific biological signaling from physics,” Scientific reports, vol. 6, 2016, doi: 10.1038/srep22874.
- B. Fichtl, I. Silman, and M. F. Schneider, “On the physical basis of biological signaling by interface pulses,” Langmuir, vol. 34, no. 16, pp. 4914–4919, 2018, doi: 10.1021/acs.langmuir.7b01613.
- M. F. Schneider, “Living systems approached from physical principles,” Progress in biophysics and molecular biology, vol. 162, pp. 2–25, 2021, doi: 10.1016/j.pbiomolbio.2020.10.001.
Specificity from Physics / Thermodynamics
We derived a model on specific pulse – enzyme interaction, which is fundamentally different. Here specificity does NOT derive from lock-and-key interactions but from physics. Only if strength of excitation, pulse strength and enzymatic optimum (which in turn is influenced by its microenvironment) „match“ the activity of the enzyme will raise significantly.
The pulse shifts the local state, e.g. the pH. If the shift is towards the pH-optimum of the enzyme (yellow) catalytic activity will increase. If the shift is insignificant (blue enzyme) no change will be detected. Of course, activity can also be suppressed.
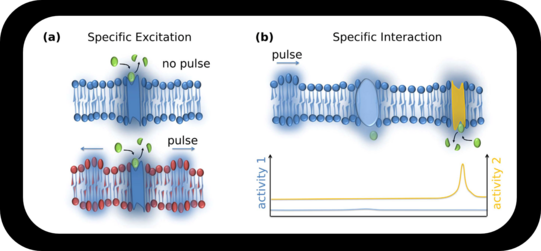
(Below). State "creates" Specificity. Assuming the system resides at some equilibrium (black dot) and is now subjected to a pulse. Only if the pulse creates thermodynamic changes which will push the system (enzyme and interface) within the transition regime (red band) the enzyme will increase its activity.
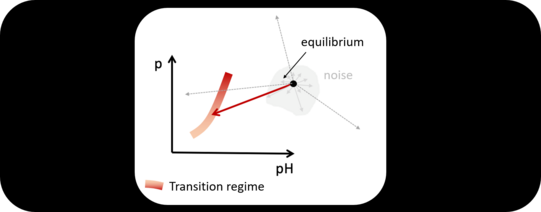
References:
- B. Fichtl, S. Shrivastava, and M. F. Schneider, “Protons at the speed of sound: predicting specific biological signaling from physics,” Scientific reports, vol. 6, 2016, doi: 10.1038/srep22874.
- M. F. Schneider, “Living systems approached from physical principles,” Progress in biophysics and molecular biology, vol. 162, pp. 2–25, 2021, doi: 10.1016/j.pbiomolbio.2020.10.001.
Action Potentials in Excitable Plant Cells
We use Characean algae as a model system for nerve pulse propagation in order to understand its physical foundation. We were able to demonstrate the annihilation of colliding pulses. Further we analyzed the phase diagram of this living system and were able to identify a physical representation of its “living state”. Only within a certain regime of physical parameters the alga remains excitable. This is the regime where nonlinear pulses occur.
References:
- C. Fillafer, A. Paeger, and M. F. Schneider, “Collision of two action potentials in a single excitable cell,” Biochimica et biophysica acta : BBA General subjects, vol. 1861, no. 12, pp. 3282–3286, 2017, doi: 10.1016/j.bbagen.2017.09.020.
- C. Fillafer, A. Paeger, and M. F. Schneider, “The living state: how cellular excitability is controlled by the thermodynamic state of the membrane,” Progress in biophysics and molecular biology, vol. 162, pp. 57–68, 2021, doi: 10.1016/j.pbiomolbio.2020.10.003
- S. Fabiunke, C. Fillafer, A. Paeger, and M. F. Schneider, “Optical studies of membrane state during action potential propagation,” Progress in biophysics and molecular biology, vol. 162, pp. 69–78, 2021, doi: 10.1016/j.pbiomolbio.2020.11.001.
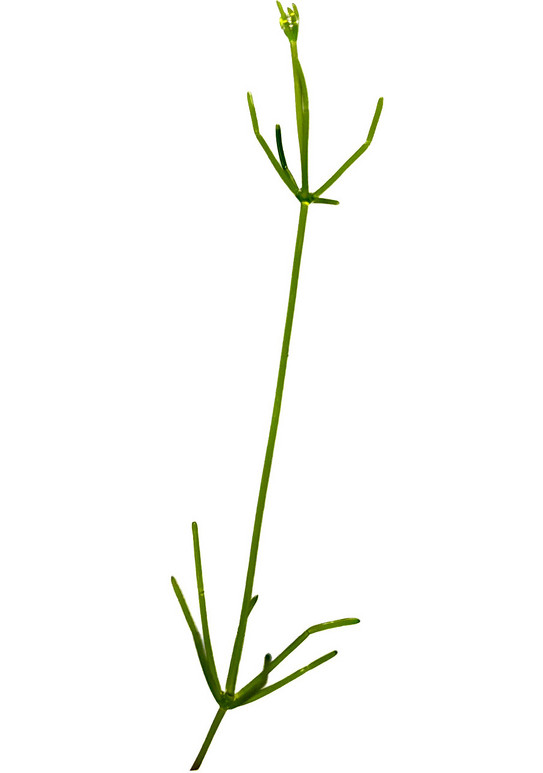
Thermodynamics in living systems
Are there traces of phase transitions in the membranes of living systems? We are doing living cell studies with intact, motile specimen of the “swimming neuron” Paramecium and fluorescence spectroscopy to observe the phase state under changing circumstances.
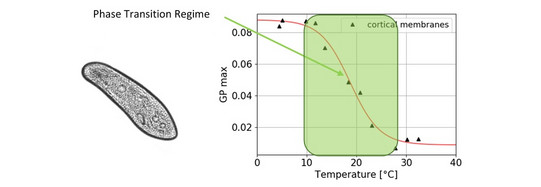
References:
- Anne Paeger, Christian Fillafer, and Matthias F. Schneider. “Evidence for a transition in the cortical membranes of Paramecium”. In: Biochimica et Biophysica Acta (BBA)-Biomembranes 1865 (1 2023), pp. 1–7. issn: 00052736. doi: doi.org/10.1016/j.bbamem.2022.184073.
- Lukas G. Schnitzler, Anne Paeger, Manuel S. Brugger, Matthias F. Schneider, and Christoph Westerhausen. “Reversible single cell trapping of Paramecium caudatum to correlate swimming behavior and membrane state”. In: Biomicrofluidics 16 (2022). doi: doi.org/10.1063/5.0084084.
Sensation by Living Systems
Do cells need specific proteins to respond to their environment? We explore how the plasma membrane can function as a supramolecular receptor.
References:
- Fillafer C., Koll Y.-S., Schneider M.F., Lipid Membrane State Change by Catalytic Protonation and the Implications for Synaptic Transmission, Membranes 12 (2022) 5. https://doi.org/10.3390/membranes12010005
- Fillafer C., Schneider M.F., On the excitation of action potentials by protons and its potential implications for cholinergic transmission, Protoplasma 253 (2016) 357-365., https://doi.org/10.1007/s00709-015-0815-4
- Fillafer C., Paeger A., Schneider M.F., The living state: How cellular excitability is controlled by the thermodynamic state of the membrane, Prog. Biophys. Mol. Biol. 162 (2021) 57-68. https://doi.org/10.1016/j.pbiomolbio.2020.10.003
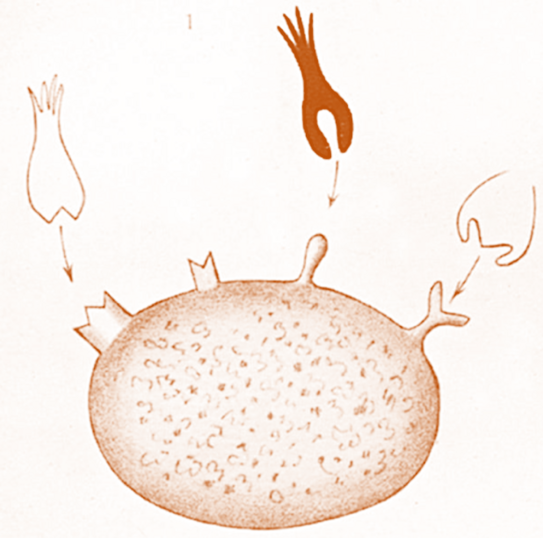
Acoustic Packets on a Flatland: A new basis for biological signaling?
This study provides first evidence that packets of sound can propagate without dispersion (solitary waves) in 2D single molecule thin films of phospholipids. The resulting systematic reorientation and condensation of the molecules was observed optically via energy transfer. The study predicts that single bio-molecules, the “inhabitants” of the flatland, can literally “talk” via the continuous 2D interface.
While the 2D acoustic phenomenon exhibit striking similarities (solitary, biphasic with a threshold) to communication in nerves, if it can indeed form a new basis for biological signaling remains to be seen.
References:
- Shrivastava S. and M. F. Schneider. Evidence for 2D Solitary Sound Waves in a Lipid Controlled Interface and its Biological Implications. J. R. Soc. Interface, 11, 97, 20140098 (2014).
- Shrivastava, S., K. H. Kang, and M. F. Schneider. Solitary Shock Waves near Phase Transition in Lipid Interfaces and Nerves. Phys. Rev. E 91, 012715 (2015).
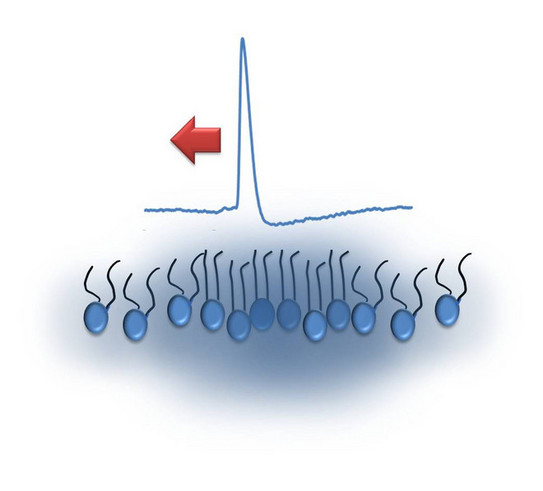
Physics of Signaling
The existence and propagation of acoustic pressure pulses on lipid monolayers at the air-water interface are directly observed by simple mechanical detection. The pulses are excited by small amounts of solvents added to the monolayer. Controlling the state of the lipid interface, we show that the pulses propagate at velocities c following the lateral compressibility κ. This is manifested by a pronounced minimum in c (∼0.3 m/s) within the transition regime. The role of interface density pulses in biology is discussed, in particular, in the context of communicating localized alterations in protein function (signaling) and nerve pulse propagation.

References:
- Fichtl B., I. Silman, and M. F. Schneider. On the physical basis of biological signaling by interface pulses. Langmuir, 34, 16, 4914-4919 (2018).
- Fichtl, B., S. Shrivastava and M. F. Schneider. Protons at the speed of sound: Predicting Specific biological signaling from physics. Sci. Rep. 6, 22874 (2016).
Synaptic transmission by acetylcholine
The transmission of a nerve pulse from a presynaptic to a postsynaptic cell (e.g., nerve, muscle, secretory cell, etc.) is fundamental for many physiological functions. In a prominent class of synapses transmission relies on acetylcholine (ACh). At present, it is assumed that ACh is (1) released by a nerve pulse, (2) binds to a membrane protein and (3) is hydrolyzed (i.e. deactivated) by acetylcholinesterase (AChE).
There are reasons to doubt this model: First, AChE is one of the fastest enzymes in nature. As such it will rapidly reduce the amount of synaptic ACh that can bind to another protein. Second, hydrolysis of ACh generates acetic acid. The protons donated by the acid may directly excite the postsynaptic membrane (as proposed by Konrad Kaufmann (Kaufmann 1977a, b, 1980)). We try to address the fundamental problem: Is a postsynaptic cell excited by intact ACh or by one of its hydrolysis products: the proton.
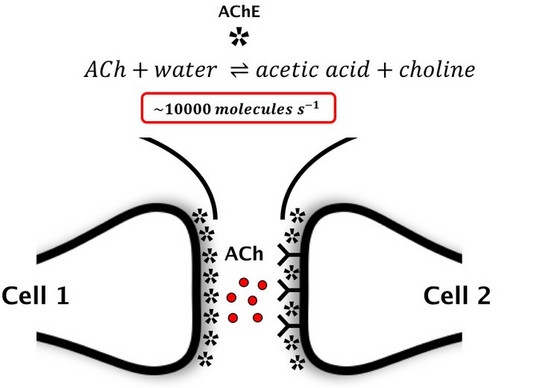
References:
- Kaufmann K., Acetylcholinesterase und die physikalischen Grundlagen der Nervenerregung (1980)
- Kaufmann K., Int. J. Quant. Chem. Supp. (1977) XII:169.
- Kaufmann K., Silman I., Naturwissenschaften (1980) 67:608.
- Fillafer C., Schneider M. F., Protoplasma (2015)
The dynamic, reversible clot
High shear induces aggregates that are only stable under flow. Once hydrodynamic stress is released they fall apart and are ready to be used somewhere else again.
Reference:
- Chen, H., M. A. Fallah, V. Huck, J. I. Angerer, A. J. Reininger, S. W. Schneider, M. F. Schneider and A. Alexander-Katz. Blood-Clotting Inspired Reversible Polymer-Colloid Composite Assembly in Flow. Nature Comm. (4) 1333 (2013).
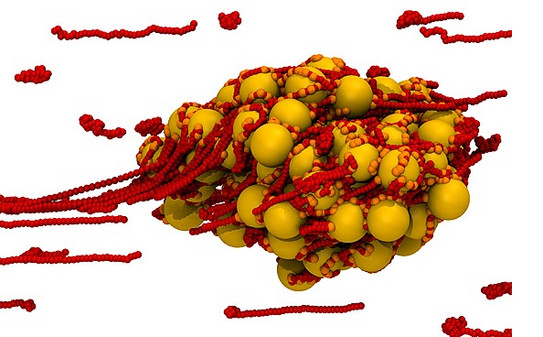